Nanotechnology Session:
Friday, September, 30th, 2022: 01:00 pm
Chairs: Wolfgang Fritzsche and Julia Böke
The session deals with micro- and nanosized particles and their investigation. On one hand, microfluidic platforms can be used to produce artificial cells, studying the cellular phenomena of self-organisation and confinement of reactions.
On the other side, micro- and nanosized particles can have a negative impact on organisms: The last years witnessed a growing awareness of the presence and potential negative impact of small plastic particles. Methods are required in order to detect and characterize these particles, preferably in a higher throughput – here aspects of methods established in cytometry could play a role. An introduction into the field will be given, and analytical approaches discussed with a special focus on a combination of imaging and flow through characterization. The potential of additional information from (spectroscopic) imaging will be discussed.

High-throughput microfluidic production of multi-compartment synthetic eukaryotic cells
Max Planck Institute of Colloids and Interfaces, Berlin
Abstract
Bottom-up synthetic biology aims at the de novo construction of synthetic cells using non-living components. Building biomimetic cells provides the opportunity to understand real cells and their origins, but also provides alternative routes to novel biotechnologies. Giant unilamellar vesicles (GUVs) are used extensively to construct synthetic cells but traditional methods to form them are limited. Microfluidic-based approaches for GUV production show great potential for encapsulating large biomolecules required for mimicking life-like functions (Yandrapalli et al. Micromachines, 2020; Love et al. Angew Chemie, 2020). First, we present a microfluidic design that is able to produce surfactant-free pure-lipid GUVs in high-throughput (Yandrapalli et al. Commun Chem, 2021). The major advancement is that the lipid membranes are produced in the absence of block co-polymers or surfactants that can affect their biocompatibility – which is commonly overlooked. The design can produce homogenously sized GUVs with tuneable diameters from 10-130 µm. Encapsulation is uniform and we show that the membranes are oil-free by measuring the diffusion of lipids via FRAP measurements. Next, we encapsulate two sub-populations of nano-sized vesicles to establish enzymatic cascade reactions across membrane-bound compartments, therefore mimicking eukaryotes (Shetty et al. ACS Nano, 2021). The final synthetic cell comprises three coupled enzymatic reactions, which propagate across three separate compartments in a specific direction due to size-selective membranes pores. Not only does microfluidics provide a high-degree of control over the intra-vesicular conditions such as enzyme concentrations, buffers, and the number of compartments, but the monodispersity allows us to directly compare the effects that compartmentalization has on the biochemical reaction rates.
Biosketch
Tom Robinson received an MSci in Physics (2005), an MRes in Chemical Biology (2007) and a PhD in Chemistry/Physics (2011) from Imperial College London. During his doctoral studies he was introduced to microfluidics under the guidance of Professor Andrew de Mello. He worked as a postdoctoral fellow at ETH Zurich with Professor Petra Dittrich from 2011 to 2014 where he developed microfluidic technologies dedicated to handling and analysing lipid vesicles. In 2014 he joined the Max Planck Institute of Colloids and Interfaces in Germany as a postdoctoral fellow with Dr. Rumiana Dimova and in 2016 he became an independent group leader within the MaxSynBio research network. His current research interests are focussed on developing microfluidics for bottom-up synthetic biology applications. In particular, his work focusses on platforms designed to both create and analyse giant unilamellar vesicles (GUVs) and their subsequent use as artificial cells. These synthetic cells are created with multiple internal membrane-bound compartments mimicking eukaryotic cell organelles. The aim is to use these multi-compartment systems to setup enzyme-mediated cascade reactions and spatial organisation of the internal structures.
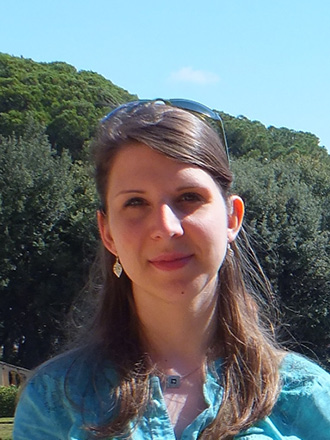
Microplastics – Small particles, big challenges
Aalborg University, Department of the Built Environment, Denmark
Abstract
With global plastic production inexorable on the rise, the occurrence of plastic pollution in the environment has been acknowledged as an emerging topic of international concern. This plastic litter is derived mainly from the inadequate disposal and management of plastic waste and will, due to physical and chemical weathering, get brittle and release a multitude of smaller and smaller plastic particles termed microplastics (1 μm – 5 mm) and nanoplastics (<1 μm).
In recent years, this microplastic pollution has received increasing attention not only from the scientific community but also from society and policymakers. However, its extent and the resulting impacts on the aquatic environment and human health remain largely unknown. This is especially true regarding the conclusive identification and characterization of “small” microplastics. The smaller the particles, the bigger the challenges related to extracting and identifying microplastics in complex environmental matrices. Furthermore, various methods for sampling, extraction, and analysis of microplastics are available, which complicate the establishment of standardized operational protocols (SOPs). So far, no single analytical method exists that can provide reliable identification and quantification of all microplastic polymers and simultaneously assess desired characteristics, e.g., size, shape, and mass.
Light and, more recently, fluorescence microscopy are widely used in microplastic research to visualize and characterize microplastics. Microscopy can be combined with spectroscopic techniques, like Fourier Transform Infrared (FT-IR) and Raman spectrometry to assess also the chemical composition. Thermoanalytical methods and mass spectrometry further provide relevant information regarding the polymers’ mass and composition, including additives.
Historically, flow cytometry was used to enumerate and characterize cells but has long since broadened in scope and is now applied for all microparticles, including microplastics. Although only a few studies have explored the possibility of utilizing flow cytometry for the quantification and characterization of microplastics in environmental samples, flow cytometry has become a helpful tool in the field of microplastic research for, e.g., the validation and quality assurance of digestion protocols, aiding in recovery experiments, and setup of ecotoxicological experiments.
Some of these applications will be presented here with a focus on the use of the FlowCam® (Yogogawa Fluid Imaging Technologies), an imaging particle analyzer combining the benefits of flow cytometry, microscopy, and digital imaging. This instrument is used worldwide for quality control for (bio)pharmaceutical products, water quality monitoring, counting cells of cyanobacteria and other marine and freshwater plankton organisms, and microplastics research.
Biosketch
Claudia Lorenz is a PostDoc at Aalborg University in the Department of the Built Environment. She is a Marine Biologist and has been working in the interdisciplinary field of microplastic research since 2014. During her PhD she was working at the Alfred Wegener Institute Helmholtz Centre for Polar and Marine Research on Helgoland investigating the spatial distribution of microplastics in the North Sea. She received a PhD scholarship from the Deutsche Bundesstiftung Umwelt (DBU) and got her PhD in Biology from Jacobs University Bremen.
Her research focus has been on optimization of sample preparation and analysis of microplastics in various environmental matrices where imaging of fluids also plays a role. Further research interests include processes driving the transport, fate, and biological interactions of microplastics as well as other aquatic pollutants.
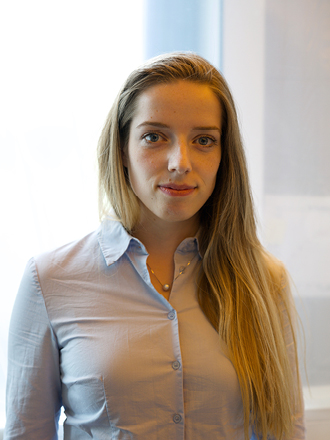
Imaging Flow Cytometry – from 3D tomographic over multispectral imaging to nanoparticle tracking
Leibniz Institute of Photonic Technology, Jena
Abstract
Particles constitute the basic building blocks of cells and tissues, which form living matter, organisms and biosystems. They are omnipresent in daily life and the environment spreading over fields like nutrition, agriculture, sustainability, waste and technology. The gold standard for analyzing large particle populations is flow cytometry (FC). It captures forward and side scattering or the fluorescent signal of each measured particle through a point detector, without resolving the spatial distribution of biomarkers or internal structures. Conventional imaging flow cytometry (IFC) bridges this gap by combing flow cytometry with an imaging setup. In our group, we combine fluid dynamics, microsystems technology, photonics and automation to develop microfluidic devices for applications ranging from microfluidic design automation over biotechnology process monitoring to motion analysis. These efforts let us develop a unique IFC platform (Figure 1) utilizing a smart combination of flow focusing and flow rotation (Henkel et al. 2020). The IFC platform enables applications in the field of tomographic IFC (tIFC) (Kleiber et al. 2020), volumetric image-based absorbance imaging, multispectral IFC (mIFC) (Kraus et al. 2022) and even Brownian motion imaging flow cytometry (bmIFC) for the analysis of nanoparticles and liposomes. Thus, it captures different particle properties, ranging from morphological to spectral characteristics, in high-throughput. Combined with a high-speed camera and suitable optics, the setup can be tailored to a variety of applications to address fundamental research questions on nanoparticles or enable continuous monitoring of cells or particles for different process parameters.
Biosketch
Julia Sophie Böke is pursuing a Ph.D. in multispectral imaging flow cytometry at the Leibniz Institute of Photonic Technology in the Microfluidics Research Group of the Nanobiophotonics department. In her research she combines spectrally resolved imaging with high-throughput microfluidics to analyze microparticles.
In 2017 she was awarded the SPIE Educational Scholarship. She received her bachelor’s degree from the Technical University of Braunschweig, Germany, in electrical engineering and business management in 2018. Julia worked as a research assistant at the Institute of High Frequency Technology, the Institute of Information Management and the Institute of Semiconductor Technology. In 2020 she successfully graduated from the European Master of Science in Photonics program as a joined degree from both Ghent University and Vrije University Brussel in Belgium.
She is currently awarded by the European Commission with the Marie Skłodowska Curie Fellowship (MSCA) for her Ph.D. project in the framework of the International Training Network MONPLAS.
Martin Hussels (Short Talk)
Physikalisch-Technische Bundesanstalt (PTB), Berlin, Germany
Integration and Control of Low-Cost Industrial Cameras in a Home-Built Flow Cytometer
Imaging flow cytometry combines the high information content of microscopic images with the high throughput of flow cytometry. Possible applications include the diagnosis of acute myeloid and lymphoid leukemia in immunophenotyping, detection of rare circulating tumor cells in liquid biopsy, or analysis of morphological cell changes like mitosis. In these applications, very small populations of target cells must be identified and quantified within huge numbers of other cells, coincidences, and agglomerates. Minor errors in counting those populations can have a major impact on the diagnosis.
As differentiation between single cells, coincidences, and cell agglomerates is challenging for common flow cytometers, we developed a flow cytometer with integrated multi-dimensional imaging. Our instrument combines the high signal detection rates of photomultiplier tubes (PMTs) with the ability to capture images of cells of interest.